Grame Davis, PhD
Graeme Davis, PhD, is the Morris Herzstein Distinguished Professor of Medicine at UCSF. Davis and his laboratory study how the brain achieves both stability and capacity for change throughout life.
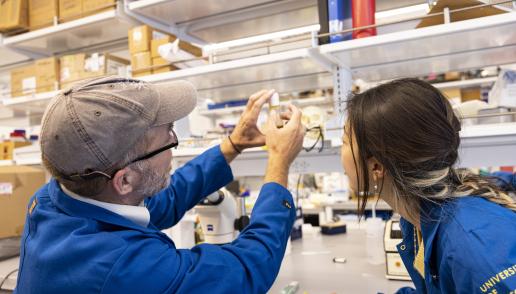
Brain Biology – constantly changing just to stay the same.
When my family gathers at my parent’s house, we will often sit down to watch old home videos – cringeworthy fun. At a recent showing, I was suddenly struck by something that is both completely obvious and an absolute mystery. Every member of my family and all my childhood friends remain completely recognizable in movies that were created twenty years ago. My friends and I were only eight years old. Yet, our characteristic facial expressions, mannerisms and attitudes are all clearly recognizable. The movies project younger reflections of our current selves. I still relate to my childhood friends in the same way. I still give my mom that same eye-roll. Each of us has certainly changed. Yet, there is an underlying, undeniable stability regarding who we were and who we remain today. What stabilizes our individuality – throughout life ?
As a member of the Kavli Institute for Fundamental Neuroscience (KIFN) at the University of California, San Francisco, I am learning about brain stability, talking with Dr. Graeme Davis, professor of biochemistry and neuroscience and director of KIFN. Davis emphasizes that, “Every aspect of our individuality and our daily life is controlled by the complex circuitry of the human brain.” This allows me to pose my question more precisely. How can the human brain achieve both stability (our life-long individuality) while simultaneously changing every single day, enabling our ability to learn about the world and adapt to new circumstances?
An entire subfield of modern neuroscience, pioneered by Dr. Graeme Davis, seeks answers to my question. This area of brain research is termed Homeostatic Plasticity. Davis points out that the term Homeostatic Plasticity encompasses concepts related to both stability and change. The word ‘Homeostasis’ refers to the maintenance of a biological process within a normal operational range. This term was first used by a famous physiologist named Walter Cannon who worked at Harvard University in the early 1900s. He was referring to, among other things, the stability of human heart rate - which increases when we exercise, but returns to a characteristic, stable resting rate. By contrast, the word ‘Plasticity’ describes how neurons can change their form and function during, for example, learning and memory. When the two terms are combined, ‘Homeostatic Plasticity’ refers to how nerve cells can make corrective, adaptive changes, stabilizing their function within a normal operational range. In other words, homeostatic plasticity refers to how nerve cells change in order to remain the same. And, like the brain itself, the way that this is achieved is very complicated.
If this sounds fascinating – read on!
Pre-explainer: The Science
The human brain is a complex network of cells (neurons) and their connections (synapses). There are an estimated 82 billion neurons in the human brain and there are hundreds of trillions of synapses. In general, new neurons stop being manufactured while you are a child. As a result, the neurons that you are born with must last your entire life. But, as Davis points out, “Neurons are constructed from the squishy, impermanent building blocks of life – water, lipids and protein. Durability is achieved by constantly updating the sub-cellular components within each of the 82 billion neurons in your brain.” This is a tremendously complex task! Neurons are enormous cells, with large tree-like arbors that fan out within the brain, the sites where synapses reside. A single neuron can receive 10,000 synapses, each of which is composed of hundreds of different proteins and different types of lipids. Adding more complexity, the unique electrical properties of each neuron is determined by tens of thousands of ion channel proteins that decorate the cell surface. And, this is just a glimpse of overall cellular complexity. Davis argues that homeostatic plasticity is related to the mechanisms that constantly update and replace proteins at synapses, as well as all those ion channels. Nerve injury (brain trauma from a concussion), environmental insult (toxins) or diseases like Alzheimer’s will induce homeostatic repair mechanisms in a manner that is similar to the normal maintenance of neuronal health. “It is the biology of nerve cell resilience”.
Now, consider that the process of learning and remembering involves changing the structure and function of individual nerve cells and synapses. This itself is remarkable, but it also makes homeostatic plasticity even more challenging to achieve. Learning-related changes must be maintained by the same processes that constantly update, repair and maintain nerve cells. So, the mechanisms of homeostatic plasticity are not as simple as manufacturing biological materials, shipping them out to distant parts of a neuron and replacing damaged components. The repairs must be precise! If not, then the events we learn would be gradually erased. It would be impossible for me to recognize my younger self in our family home videos. So, homeostatic plasticity is a flexible, dynamic, accurate neuronal repair mechanism. And, it is uniquely important to the brain because our nerve cells must persist throughout life.
How do you study Homeostatic Plasticity?
“In order to study stability, you need to break things and determine what happens next.”
Twenty-five years ago, Davis began his search for genes that are involved in homeostatic plasticity. Where do you start when absolutely nothing is known? Davis is emphatic, “It is a fool’s errand to make guesses about how things work in biology.” Instead, Davis set out to test the function of every gene in the genome, one by one, and determine which ones are important for homeostatic plasticity.
How long does it take to test each gene? Davis developed an assay in the early 2000’s, a summer project that he conducted himself. He was studying the connection between nerve and muscle, termed a ‘synapse’. Synapses are sites of communication between cells in the brain, and between nerve and muscle. Davis discovered a toxin that partially impaired synaptic communication. He applied the toxin and function was immediately disrupted. But, he discovered that if he waited half an hour, function was restored even though the toxin was still present and still active. Further work demonstrated that the synapse had changed in the presence of the toxin, adjusting specific parameters to overcome the effects of the toxin and restore normal communication between nerve and muscle. This was evidence of homeostatic plasticity. The assay takes about an hour, start to finish, and must be repeated several times for each gene that is being tested. A hard working scientist can assay 2 genes in a day. Over the past 20 years, this assay has been repeated more than 15,000 times. He estimates that they have tested nearly half of the genes in the genome, one by one. This is slow, repetitive, difficult and often boring work. Sometimes, individuals work every day and fail to find a new gene for an entire year. But, the end result is fantastic: “We have discovered genes that we could never have guessed might be involved. It is new biology. And, this is the fuel that will allow us to develop therapies to promote resilience to brain injury and disease.”
Davis is quick to point out that students in his lab are not simply laboring away, discovering gene after gene. “It is not an assembly line!” When we find a gene, it opens the door to a host of questions that we all grapple with. How do neurons detect that they have been perturbed? How is homeostatic plasticity regulated so that it does not over-compensate and cause harm? If we prevent homeostatic plasticity, will it alter disease? Students can tackle the questions that they find most interesting, using their new found gene(s) as tools to probe and prod how homeostatic plasticity works in the context of health and disease. “Experimental biology requires patience, persistence and endurance, but it is absolutely fascinating!”
Will this improve human health?
“We might even delay the insidious, inevitable, adverse effects of advancing age.”
Is homeostatic plasticity some esoteric area of brain science, or is it relevant to brain disorders and human disease? As Davis emphasizes, there are powerful, endogenous mechanisms that are always at work to maintain brain stability – homeostatic plasticity. The Davis lab has been on a mission for the past 25 years to define the underlying molecular mechanisms of Homeostatic Plasticity. “If we can identify the genes and molecules that achieve homeostatic plasticity, then we may be able to enhance the process and improve brain resilience[MOU1] [MOU2] . This could have tremendous value. We still do not know the underlying cause of most neurodegenerative diseases or psychiatric disorders. Causes are likely to be a complex mixture of gene malfunction and environmental insult. As a result, we have very few effective therapeutics. But, if we could promote brain resilience, then the underlying cause of disease is irrelevant. We would improve the ability of nerve cells to resist diseases like Alzheimer’s Disease and amyotrophic lateral sclerosis (ALS), regardless of the underlying cause. We might even delay the insidious, inevitable, adverse effects of advancing age.” In a recent publication, Davis established a new model for how homeostatic plasticity opposes the onset and progression of ALS in mice. In doing so, he coined the term “Homeostatic Neuroprotection” to emphasize how homeostatic plasticity protects against brain disease.
Could too much of a good thing become a problem? Some scientists question whether promoting homeostatic plasticity will somehow prevent the cellular changes that are necessary for our ability to learn and remember. Davis considers this unlikely. In fact, he suggest that the opposite may be true. In a review article published in 2013 in the journal Neuron, Davis wrote, “Without mechanism to ensure robust, reproducible baseline neural function, our ability to learn and remember would be lost in the chaos of daily experience dependent change.” In other words, homeostatic plasticity likely facilitates learning instead of acting to oppose it. Simply put, it is easier to pay attention to new information in a quiet classroom as opposed to a crowded, noisy bar.
Biography: How did you become a scientist?
Biography: How did you become a scientist?
Davis grew up in a family where academic life was highly valued. Both his parents were high-school teachers working at a progressive boarding school in New England. But, academic science was not an early focus. When Davis went to college, he planned to study literature, hoping to one day become an English professor at a four year college. He switched to neuroscience almost by accident. He took a class in animal physiology that led to a summer job. His college mentor, Dr. Steve Zottoli, took Davis to the Marine Biological Laboratory in Woods Hole, Massachusetts for a summer research experience. This changed everything. Davis found himself surrounded by scientists of all ages, deeply engaged in their research, all doing their own experiments. Scientists worked around the clock, but did so because they were driven to answer their own questions and produce meaningful advances. According to Davis it was completely different than laboratory courses in high school or college where you follow a recipe and hope to get the correct answer. Lab reports in college are formulaic exercises. By contrast, that summer job in Woods Hole was the moment that Davis realized that scientific research is boundless. Questions need to be formulated. No one tells you what to do. Answers are unknown and data are the only currency for progress. This seemed thrilling. At the same time, Davis also noted that the oldest scientists engaged in their research as avidly as the youngest students. “I clearly recall thinking (I was only 20 yrs old at the time) that this was how I wanted to end up…still excited and engaged with the world in my 70’s.” Davis majored in Biology and subsequently attained a PhD in Neuroscience and Behavior from the University of Massachusetts. Then, Davis pursued postdoctoral work at the University of California in Berkeley where he learned genetics, molecular biology and discovered the first evidence of homeostatic plasticity. Davis started his own laboratory at the University of California in San Francisco in 1998 as an Assistant Professor. He has been pioneering our understanding of how homeostatic plasticity stabilizes the brain in health and disease ever since. He is now the Morris Hertzstein Distinguished Professor of Medicine, Director of the Kavli Institute for Fundamental Neuroscience and a member of the Department of Biochemistry and Biophysics at UCSF.